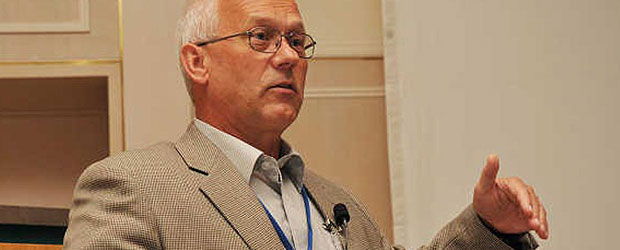
The photonics revolution at the beginning of the XXI century has its roots in the spectacular development of semiconductor devices based on quantum well (QW) microstructures that became the enabling force in constructing active photonics devices. In comparison with Si-based electronic integrated-circuit Complementary Metal-Oxide Semiconductor (CMOS) technology, the fabrication of photonic devices has no single-platform integration technology available that would be capable of providing global manufacturing solutions. Consequently, integrated photonic and optoelectronic circuits, which require different bandgap materials, have been traditionally fabricated using various hybrid manufacturing schemes. The fabrication of Monolithically Integrated Photonic Circuits (originating from the same s
emiconductor wafer) has been less successful and only limited examples of such structures have been demonstrated to date.It is widely recognized that further progress of photonics-based telecommunication and life science sectors will be related to novel concepts of using photons for increased data transfer, manipulation, storage, detection, diagnostics and therapeutics. On the device level, the task of increasing the number of different operations performed by a photonic device in an ever-shrinking volume will require nanoscale manufacturing precision. More importantly, such precision becomes a must when increased or new device functionality is expected from QW microstructures that have their optical properties (bandgap structure) modified selectively within a single semiconductor wafer. This also applies to even more technologically challenging quantum semiconductors, such as quantum dots (QDs), quantum wires, nanoclusters, and various nanocomposites. In spite of the spectacular progress in this field, present-day technologies of semiconductor materials and devices are far from adequate to meet the challenge of nanoscale manufacturing precision of photonic devices. Thus, new enabling technologies and processing schemes have to be developed to address this issue.
Research Topics
Our program ‘Laser-based Nanotechnology: Monolithically Integrated Photonics, QD Fabrication/Tuning, BioNanophotonic Devices’ addresses both fundamental and practical aspects of knowledge concerning quantum semiconductors (QS) and innovative technologies for the fabrication of QS materials and related bio-nanophotonic devices.
Quantum Semiconductor Biosensors
This research aims at developing a quantum semiconductor-based biosensor for the rapid detection and typing of human viral pathogens. One axis of the research concerns so-called epitaxial quantum dot biosensor (eQD), while this second axis is defined by a monolithically integrated quantum well surface plasmon resonance (QW-SPR) biosensor. Our initial focus is on the Influenza A biosensor, but other human pathogens will be addressed with the eQD platform technology. The goal is to develop a commercially viable (cost-effective) eQD device for the rapid detection and typing of human viral and bacterial pathogens.
The objective of this research is to investigate both fundamental and applied aspects of laser-based processes of quantum well and quantum dot intermixing that have the potential to lead to cost-attractive fabrication of advanced photonic devices or monolithically integrated photonic circuits that otherwise would have not been attainable with conventional micro-/nanofabrication methods.


The work function of n-alkanethiol self-assembled monolayers (SAMs) prepared on the GaAs(001) surface was measured using the Kevin Probe technique yielding the SAM 2D dipole layer potential (DLP). Direct n-dependent proportionality between the DLP values and the C-H band infrared (IR) absorption intensities was observed, supporting a correspondence of reported IR enhancements with the electrostatic properties of the interface. X-ray photoelectron spectroscopy is also used to reinforce the work function measurements. In addition, the principal components of the refractive index are shown to be n-invariant in the ordered SAM phase. Our results support that a local field correction to the transition dipole moment accounts for the observed increase in IR activity.